Revolution in Imaging Tech Brings Heart Failure Molecule Into View
With the latest in high-resolution scientific cameras, scientists are, for the first time, getting a clear picture of a molecule linked to heart disease and muscle diseases
Using the same technology that made smartphone cameras possible, scientists at Columbia University Medical Center are capturing images of individual molecules at a level of detail never before possible—including images of a molecule implicated in heart disease and muscle diseases.
The new images of the ryanodine receptor—a membrane protein that functions as a channel for the passage of calcium ions in muscle cells —have led to the determination of its 3-D structure. Defects in the ryanodine receptor can lead to heart failure, age-related muscle weakness, and muscular dystrophy.
The newly discovered structure will make it possible for researchers to understand how the molecule works, why it fails in heart disease, and how to design drugs to improve the function of faulty receptors.
These results are the product of close collaboration among the laboratories of Andrew Marks, MD, who has studied the physiology of ryanodine receptors for many years; Joachim Frank, PhD, who is a world-leading expert in electron microscopy; and Wayne Hendrickson, PhD, who is an eminent X-ray crystallographer.
Camera advances spark renaissance in molecular imaging
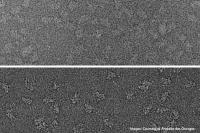
Biological molecules are tiny, with even the largest molecules only 1/10,000th as large as the width of a human hair.
The highest-resolution images of such molecules have traditionally been captured with a technique using X-ray diffraction from precisely arranged crystals of the molecule of interest. Unfortunately, making crystals of many of the biomolecules of most interest to biologists and pharmaceutical companies is challenging and, for some, nearly impossible.
A wider variety of biomolecules can be imaged with electron microscopy, which does not require crystals. But until the advent of new cameras, the resolution of such images was often too low to obtain precise models of the molecules with the exquisite details needed by drug designers.
One of the first of the new cameras was deployed in 2012 in the lab of Frank, professor of biochemistry and molecular biophysics and of biological sciences at Columbia and an HHMI investigator, who developed the approach of determining the structure of molecules from many single images.
Like its smartphone counterparts, the camera is based on active pixel sensor technology that provides superior resolution, higher contrast, and fewer image artifacts than the charged couple device-based cameras used previously.
Cameras in phones first became available in the early 2000s, but cameras for molecular imaging took longer because of the electron microscope that serves as the “lens.” Electron microscopes capture images by bombarding molecules with electrons, which would damage sensors designed to detect less-energetic photons.
“Once engineers were able to devise sensors that can withstand electrons, the first cameras came on the market soon after, and they have sparked a renaissance in molecular imaging,” Frank says.
To obtain images of the ryanodine receptor, Ran Zalk, a postdoctoral fellow in the lab of Marks, the Clyde and Helen Wu Professor of Molecular Cardiology and chair of physiology and cellular biophysics at Columbia, first prepared a purified solution containing billions of copies of the molecule. The sample was applied to a flat surface (a grid) and flash frozen to keep the molecules stationary.
The grid was then placed in the electron microscope and electrons were shot through the sample. When the electrons bounced off the molecules and passed through the lenses, they landed on the camera sensor, producing “projections” that could be transformed into images of the molecules.
Using image-processing techniques to average the projections together, Amédée Des Georges, a postdoctoral fellow in the Frank lab, created a 3-D model of the molecule.
Technique reveals never-before-seen details of ryanodine receptor
When the ryanodine receptor channel works correctly, the molecule regulates contractions in heart and skeletal muscle by controlling the passage of calcium ions through its central pore.
By contrast, when the ryanodine receptor channel malfunctions, it allows calcium to leak through the pore, which worsens heart failure or causes muscle weakness in Duchenne muscular dystrophy, an inherited disorder that affects one out of 3,500 boys.

Previous attempts by Frank to uncover the ryanodine receptor’s shape in the early 90s —with an older-generation electron microscope, film recording, and much less powerful computers—had revealed the general outlines of the molecule, including the pore. But they did not provide a picture of the nooks and crannies, which is necessary to understand how the molecule works and how defects in it cause disease.
The new model reveals these details, as well as some that had not been anticipated. The molecule is full of helical structures—arranged one after another—that give it a side-to-side flexibility that the Columbia team thinks may be involved in opening the calcium channel.
Images provide enough detail for atomic modeling of ryanodine receptor
Though this new image of the ryanodine receptor channel contains details down to 5 ångström (one tenth of a nanometer), additional modeling was needed to locate the position of each atom in the molecule.
Adapting techniques used in X-ray crystallography, structural biologist Oliver Clarke, a postdoctoral fellow in the lab of Hendrickson, University Professor in the Department of Biochemistry and Molecular Biophysics and Violin Family Professor of Physiology and Cellular Biophysics, was able to map each amino acid of the molecule onto the structure to create a more refined “atomic” model.
With this model, biologists can now see where mutations in the ryanodine receptor channel occur, understand how the mutations may affect the functioning of the channel, and model how the ryanodine receptor channel opens and closes.
The new model was published in the Jan. 1 issue of Nature.
More details of the ryanodine receptor channel should become clear in the next year, as the researchers work to obtain images of the molecule with its pore open (the current study reveals the channel structure only with the pore closed).
Drugs that fix the leaky, malfunctioning ryanodine receptor channel have already been developed; they improve muscle force in animals with age-related muscle weakness and Duchenne muscular dystrophy and improve heart function in animals with heart failure. Clinical trials to test the drugs in boys with Duchenne muscular dystrophy will soon begin.
“Having the structure in hand will completely change the field of ryanodine receptor channel research,” says Marks. “It will give us new insights into how the drugs work and how to make them work better.
References
The paper is titled, Structure of a mammalian ryanodine receptor. Additional authors are: Robert A Grassucci, Steven Reiken, and Filippo Mancia (Columbia University Medical Center).
The research was supported by the NIH (R01AR060037, R01HL061503, U54GM095315, R01GM29169); the Howard Hughes Medical Institute; American Heart Association (0625919T); National Health and Medical Research Council, Australia; and the Charles H. Revson Foundation.
The authors declare no competing financial interests.